EE4650 Advanced Magnetic Resonance Imaging
This course presents the principles of magnetic resonance from both a quantum and classical mechanics point-of-view. We start from the interaction of strong electromagnetic fields at high, medium and low frequencies, and how these can be manipulated to encode spatial information, leading to MRI images. We also discuss the origins of image artifacts, and the signal processing algorithms involved in image reconstruction.
Further, we discuss the relaxation phenomena associated with magnetic resonance, and how these are important in determining image contrast. We present how the different components of magnetic resonance systems are designed using Maxwell’s equations, spherical harmonics, and the Biot-Savart law.
Finally, we present the current state-of-the-art of MRI hardware and image processing, and the new opportunities for future developments.
Course contents
- General introduction, history of MRI, general description of an MR scanner, general principles of MRI, applications and current research themes.
- General and brief description of spin, restriction to particles with spin ½ (proton), equilibrium magnetization for spin ½ particles (proton), the Bloch equation, general description of the gyromagnetic ratio, T1 and T2 relaxation times.
- Electromagnetic fields: Maxwell’s equations, the electromagnetic boundary conditions, the temporal and spatial Fourier transform, time-harmonic fields, the polarization state of an electromagnetic field (linear, circular, elliptical), Poynting’s theorem (power balance), and reciprocity.
- Flipping the magnetization: received signal in terms of the electromagnetic receive field. Solutions of Bloch’s equation for various magnetic fields, the most important formula in MRI: formula for the Larmor frequency, chemical shift, scalar coupling, basics of 1-DNMR spectroscopy, elementary measurement sequences, spin-echo, inversion recovery, measuring T1 and T2, introducing T2*.
- Using gradients to spatially encode signals, k-space formalism, image reconstruction via multi-dimensional Fourier transforms, simple gradient echo and spin echo pulse sequences, three-dimensional imaging, angiography.
- Background knowledge for hardware design: Maxwell’s equations and quasi-static fields, the law of Biot-Savart, spherical harmonics. Interaction of RF with tissue as a function of frequency, different media/tissue types and the constitutive relations.
- RF coil and array design: design of RF coils and RF arrays, the Specific Absorption Rate (SAR). The electromagnetic field due to an electric dipole (small current-carrying piece of wire) and a magnetic dipole (small current-carrying loop).
- Gradient coil design and the static background field: Realizing gradient fields, Helmholtz and Maxwell coils, advanced gradient coil design using stream functions, consequences of nonuniform static background fields.
- Numerical modeling, software packages, body models, coil models.
- Measurements and imaging in practice, SNR and other practical aspects.
- Current/future research topics, parallel imaging, sparse sampling, electrical properties tomography.
Teachers
dr.ir. Rob Remis (THZ)
Electromagnetic and acoustic wavefield modeling, imaging, and inversion
prof.dr. Andrew Webb (THZ)
High field MRI and its clinical applications, Electromagnetism
Last modified: 2023-11-04
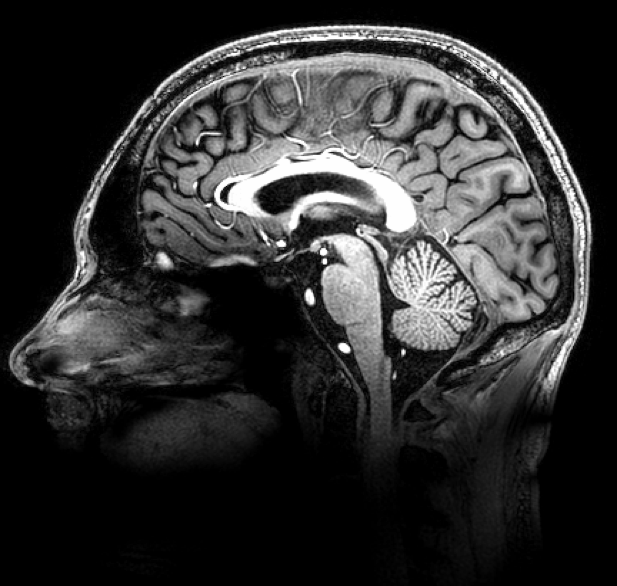
Details
Credits: | 5 EC |
---|---|
Period: | 0/0/0/4 |
Contact: | Rob Remis |